by
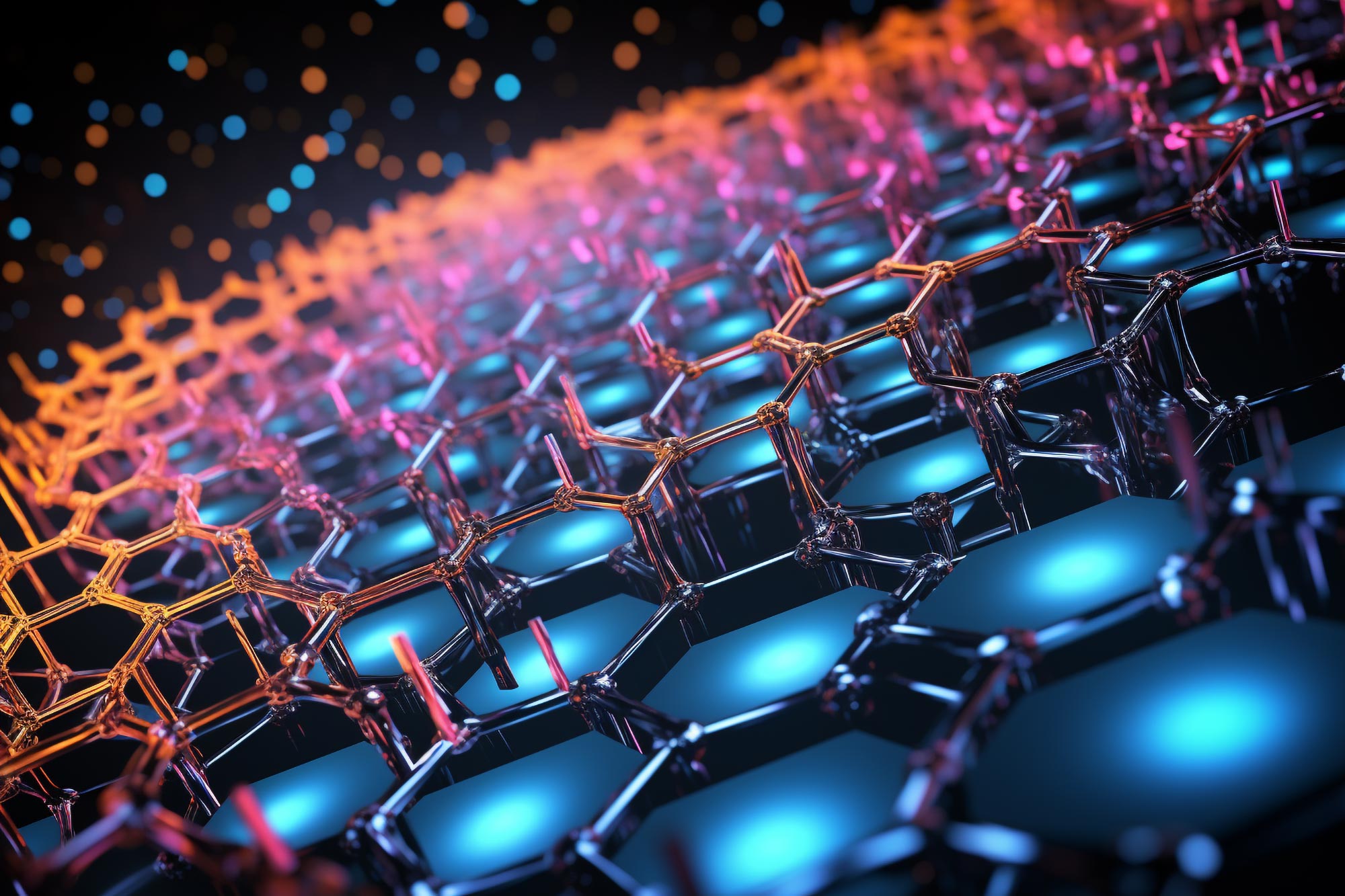
MIT researchers combine twisted electronics with quasicrystals, revealing a new way to understand superconductivity and promising applications in electronics.
A new flexible platform could create mysterious materials and lead to new studies of strange phenomena.
In research that may spark interest in a mysterious class of materials known as quasicrystals, Massachusetts Institute of Technology Scientists and colleagues have discovered a relatively simple and flexible way to create new atomically thin versions that can be tuned to fit phenomena of interest. In the work reported in the latest issue of the journal naturethey describe doing just that to make the material exhibit superconductivity and more.
The research provides a new platform not only to learn more about quasicrystals, but also to explore exotic phenomena that may be difficult to study but may lead to important applications and new physics. For example, a better understanding of superconductivity, where electrons pass through a material without resistance, could allow for more efficient electronic devices.
Image of a moiré quasicrystal (middle column) created by three overlapping sheets of atomically thin graphene. Credit: Sergio C. De la Barrera/University of Toronto
Twistronics and its association with quasicrystals
This work brings together two previously unconnected fields: quasicrystals and twisted electronics. The latter is the specialty of Pablo Jarillo Herrero, the Cecil and Ida Green Professor of Physics at MIT and corresponding author of the new book nature The breakthrough of graphene at a “magic angle” in 2018 catapulted the field.
“It is truly extraordinary that the field of chiral electronics continues to make unexpected connections with other areas of physics and chemistry, in this case the beautiful and strange world of quasperiodic crystals,” says Jarillo-Herrero, who is also affiliated with MIT’s Materials Research Laboratory and MIT. “. MIT Research Laboratory for Electronics.
Notable developments in Twistronics
Twistronics technology involves thin layers of materials layered on top of each other. Rotating or twisting one or more of the layers at a slight angle creates a unique pattern called a super moiré mesh. The moiré pattern, in turn, has an effect on the behavior of electrons. Sergio C says: “It changes the spectrum of energy levels available to electrons and could provide the conditions for interesting phenomena to emerge,” said de la Barrera, one of four co-authors of the recent study. De la Barrera, who conducted this work as a postdoc at MIT, is now an assistant professor at the University of Toronto.
Aviram Uri (left) and Sergio C. The de la Barrera are part of a team that extracted superconductivity from an obscure class of materials known as quasicrystals. Uri is a Pappalardo and postdoctoral researcher in VAT at MIT; De la Barrera is an assistant professor at the University of Toronto. Credit: Eva Cheung/University of Toronto
A moiré system can also be designed for different behaviors by varying the number of electrons added to the system. As a result, the field of chiral electronics has witnessed tremendous development over the past five years, with researchers around the world applying it to create new atomically thin quantum materials. Examples from MIT alone include:
- Converting a moire material known as a magic angle twisted bilayer Graphene into three different and useful electronic devices. (Among the scientists involved in this work, reported in 2021, was Daniel Rodin-Legren, co-first author of the current work and an MIT postdoctoral researcher in physics. They were led by Jarilo Herrero.)
- Engineering a new property, photovoltaics, into a well-known family of Semiconductors. (The scientists involved in this work, Reported in 2021led by Jarillo Herrero.)
- Predicting new and strange magnetic phenomena, complete with the “recipe” for achieving them. (The scientists involved in this work, Reported in 2023, included MIT physics professor Liang Fu and Nisarja Paul, an MIT physics graduate student. Both Fu and Paul are co-authors on the current paper.)
Unveiling quasicrystals
In the current work, the researchers were tinkering with a moiré system made of three sheets of graphene. Graphene consists of a single layer of carbon atoms arranged in hexagonal shapes resembling a honeycomb structure. In this case, the team layered three layers of graphene on top of each other, but twisted two of the sheets at slightly different angles.
To their surprise, the system created what looked like a crystal, an unusual class of material that had been discovered in the 1980s. As the name suggests, quasicrystals fall somewhere between a crystal, such as a diamond, which has a regular repeating structure, and an amorphous material, such as glass, “where the atoms are all mixed up, or randomly arranged,” says de la Barrera. In short, de la Barrera says that quasicrystals “have really strange patterns” (see some examples here).
However, compared to crystals and amorphous materials, relatively little is known about quasicrystals. This is partly because they are difficult to make. “That doesn’t mean it’s not interesting; it means we haven’t paid much attention to it, especially to its electronic properties,” says de la Barrera. The new, relatively simple platform could change that.
More insights and collaboration
Because the original researchers were not experts in quasicrystals, they reached out to one person: Professor Ron Lifshitz of Tel Aviv University. Aviram Uri, one of the paper’s co-first authors and a Pappalardo and Vatat MIT postdoctoral fellow, was a student of Lifshitz during his undergraduate studies in Tel Aviv and was aware of his work on quasicrystals. Lifshitz, who is also the author of nature The paper helped the team better understand what they were looking at, what they call quasicrystalline moiré.
Next, physicists fine-tuned the moiré quasicrystal to make it superconduct, or conduct current without any resistance at all below a certain low temperature. This is important because superconducting devices can transmit current through electronic devices much more efficiently than is possible today, but this phenomenon is still not fully understood in all cases. The new corrugated quasicrystalline system provides a new way to study it.
The team also found evidence of symmetry breaking, another phenomenon that “tells us that electrons are interacting with each other very strongly.” “As quantum physicists and materials scientists, we want our electrons to interact with each other because that’s where the exotic physics happens,” de la Barrera says.
In the end, “through discussions across continents, we were able to decipher this thing, and now we think we have a good control over what’s happening,” Urey says, although he points out that “we don’t yet fully understand the system.” . There are still quite a few mysteries.”
The best part of the research, says de la Barrera, was “solving the puzzle of what we actually made.” “We were expecting [something else]So it was a very pleasant surprise when we realized that we were actually looking at something very new and different.
“It’s the same answer for me,” Urey says.
Reference: “Superconductivity and strong interactions in a tunable moiré quasicrystal” by Aviram Uri, Sergio C. de la Barrera, and Malika T. Randrea, Daniel Rodin-Legren, Trip Devakul, Philip J.D. Crowley, Nisarja Paul, Kenji Watanabe, Takashi Taniguchi, Ron Lifshitz, Liang Fu, Raymond C. Ashuri, Pablo Jarillo Herrero, July 19, 2023, nature.
doi: 10.1038/s41586-023-06294-z
Additional authors for nature The paper is by MIT physics professor Raymond C. Ashouri; Malika T. Randrea, a researcher at MIT Lincoln Laboratory who conducted the work as a Pappalardo Fellow at MIT and is co-first author of this paper; Trithip Devakul, an assistant professor at Stanford University who conducted the work as a postdoctoral researcher at MIT; Philip J.D. Crowley, a postdoctoral researcher at Harvard University; and Kenji Watanabe and Takashi Taniguchi of the National Institute of Materials Science in Japan.
This work was funded by the US Army Research Office, the US National Science Foundation, the Gordon and Betty Moore Foundation, an MIT Pappalardo Fellowship, a VATAT Distinguished Postdoctoral Fellowship in Quantum Science and Technology, JSPS KAKENHI, and the Israel Science Foundation.
“Typical beer advocate. Future teen idol. Unapologetic tv practitioner. Music trailblazer.”
More Stories
Boeing May Not Be Able to Operate Starliner Before Space Station Is Destroyed
How did black holes get so big and so fast? The answer lies in the darkness
UNC student to become youngest woman to cross space on Blue Origin